V2G Turns Electric Vehicles Into Distributed Energy Resources
October 24, 2024
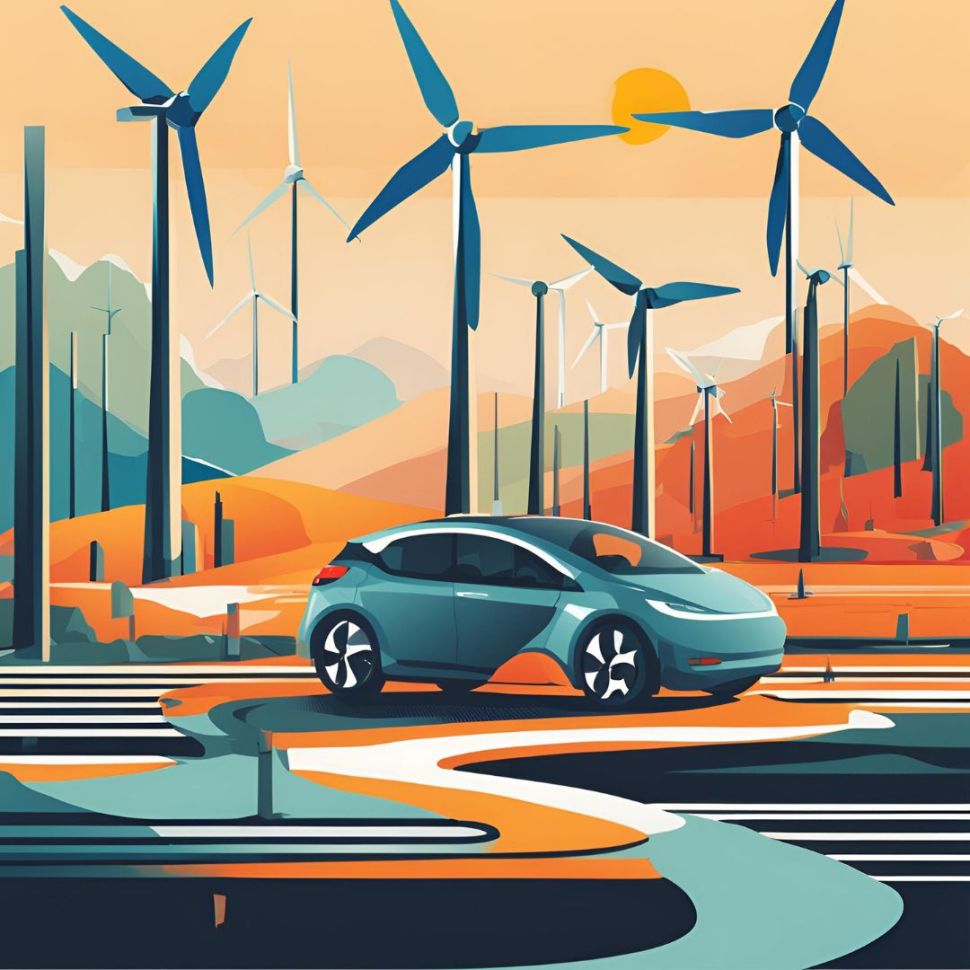
The energy transition embodies several important trends. Chief among them are the transition of the electric grid from fossil-based to renewable energy resources, and the shift from cars powered by internal combustion engines to cars powered by batteries. Both involve electricity but, to the casual eye, they may not seem to have much in common. A closer look and a back-of-the-envelope calculation suggest that, in fact, they will have a great deal to do with one another.
The legacy grid that has powered so many facets of our lives over the last hundred-plus years has many extraordinary properties. One is the promise to deliver to each of us as much electricity as we want, at the exact instant we want it, for as long as we want it, without us having to ask for it—and the fulfillment of that promise for the vast majority of the hours each year. Electric utilities have been able to deliver on the promise by building and operating collections of generating stations that are managed with precise top-down control.
By contrast, the grid of the future will be powered in substantial measure by generating technologies that are not subject to top-down control. Most conspicuously, photovoltaic generation produces only as much electricity as the sun’s position and atmospheric conditions allow; and wind power produces only as much electricity as local air currents allow. Solar and wind together account for almost 60 percent of the world’s 3.3 terawatts of non-fossil-based electricity generating capacity, which translates to approximately 25 percent of total global generating capacity of 7.9 terawatts. At that ratio, the world’s generating capacity that is “dispatchable” can be managed in a way that heads off any issues arising from the intermittent and unpredictable nature of many renewable resources.
But what will happen as the share of solar and wind grows? Looking at the jurisdictions that have already attained higher percentages of wind and solar, we see that a number of measures have been adopted. Many take the form of energy storage, wherein quantities of electricity that are generated beyond current demand are absorbed by facilities that are able to store it in a form that can be readily sent back to the grid during periods of high demand. Pumped hydro and stationary battery installations are prominent in this space. As a general rule, the levelized cost of kilowatt-hours produced by wind and solar is low relative to legacy generating sources, while the cost of kilowatt-hours that come out of storage facilities is high.
The back-of-the-envelope calculation starts with the 1.5 billion cars in our current global vehicle fleet. Today, most of these cars are powered by fossil fuels, but there is no doubt that substantial conversion to battery electric power is underway. Electric vehicles can be refueled under a variety of different charging standards. One of the most prevalent (and far from the most powerful) is so-called Level 2. The maximum rate of power delivery with Level 2 is just under 20 kilowatts. This means that if something like 28 percent of the global fleet of cars were to be converted to electric power, and then plugged into Level 2 chargers all at once, global electricity demand would double, from 7.9 to 15.8 terawatts.
This is the point of convergence between the conversion-to-renewables trend and the conversion-to-batteries trend. Whereas most electric cars today function like any other electricity-consuming appliance, i.e., draw power when they are plugged in, a select few can take electricity from their batteries and, under vehicle-to-grid (V2G) schemes, send it back to the grid. Creating this power export capability is neither difficult nor expensive. If it were to become a standard feature of most EVs, the 7.9 terawatts of additional power consumption noted above could be turned into 7.9 terawatts of power availability. In other words, this cadre of electric cars could power the global grid.
There is no scenario in which the global fleet of EVs will be called upon to power the global grid in its totality. However, having a resource of this magnitude at hand as more and more renewable generating facilities are brought online is serendipitous in several ways. First, it means that there is almost no degree of renewable generation intermittency that could not be handled by bidirectional EVs. Next is an alignment of need and availability on the energy dimension. Whereas the global fleet of EVs will foreseeably be large enough to power the global electricity grid, it could only do so for a period of time measured in hours. In most cases, though, the need will only be there for periods of time measured in hours, or even minutes—for example, when the sun is setting in a given location, the night-time wind has not yet picked up and the early-evening peak in electricity demand is arriving.
One final serendipitous circumstance is the availability of information technology that can generate and process massive, continuous data flows, and use that data to exercise automated control over large numbers of widely distributed nodes. IT, and increasingly artificial intelligence, is allowing comprehensively smart management of the grid, including monitoring and management of discrete sources of both supply and demand. This capability is essential for all forms of grid decentralization, especially when dual-purpose assets such as electric vehicles are involved. (Vehicle owners need to be able to set ground rules for the use of their cars as grid-support assets—“must be fully charged by 7:00 a.m. tomorrow”—and be confident that those rules will be rigorously followed.)
The factor that ultimately makes the case for electric vehicles as distributed energy resources is economics. As noted, dedicated electricity storage facilities are expensive. The incremental cost of a power-export-ready EV is not. Even factoring in the cost of the battery wear-and-tear that comes with delivery of grid services does not flip the equation. Vehicle owners can be compensated for the use of their batteries, reducing their total cost of ownership, and the cost to deliver grid services will still be lower than that of single-purpose storage assets.
It must be acknowledged that the integration of electrified transportation into the evolving grid has been slow. The essential concepts are simple. Putting them into practice in the real world is highly complex. The issues that must addressed range from the technical (e.g., developing industry standards to support interoperability across system nodes); to the regulatory (e.g., how to structure the permitting process for electric vehicles as grid-support resources); to the financial (e.g., how to ensure that compensation mechanisms create participation incentives for all parties in the value chain); and beyond.
Nonetheless, there is now a track record of practical progress that started with the earliest commercial vehicle-to-grid deployments in Scandinavia in the mid-2010s. Going forward, the pace of vehicle-grid integration seems poised to accelerate. For example, early this year, an electric school bus fleet in California was announced that will shortly be providing daily grid-support service with a power export capacity of more than two megawatts. (That is the equivalent of approximately 80 residential-scale backup generators.) The future in which electric transportation and the electric grid are so comprehensively intertwined that they no longer have fully distinct identities may never arrive. But at this moment, it seems more likely than not that it will.
Search
RECENT PRESS RELEASES
Related Post